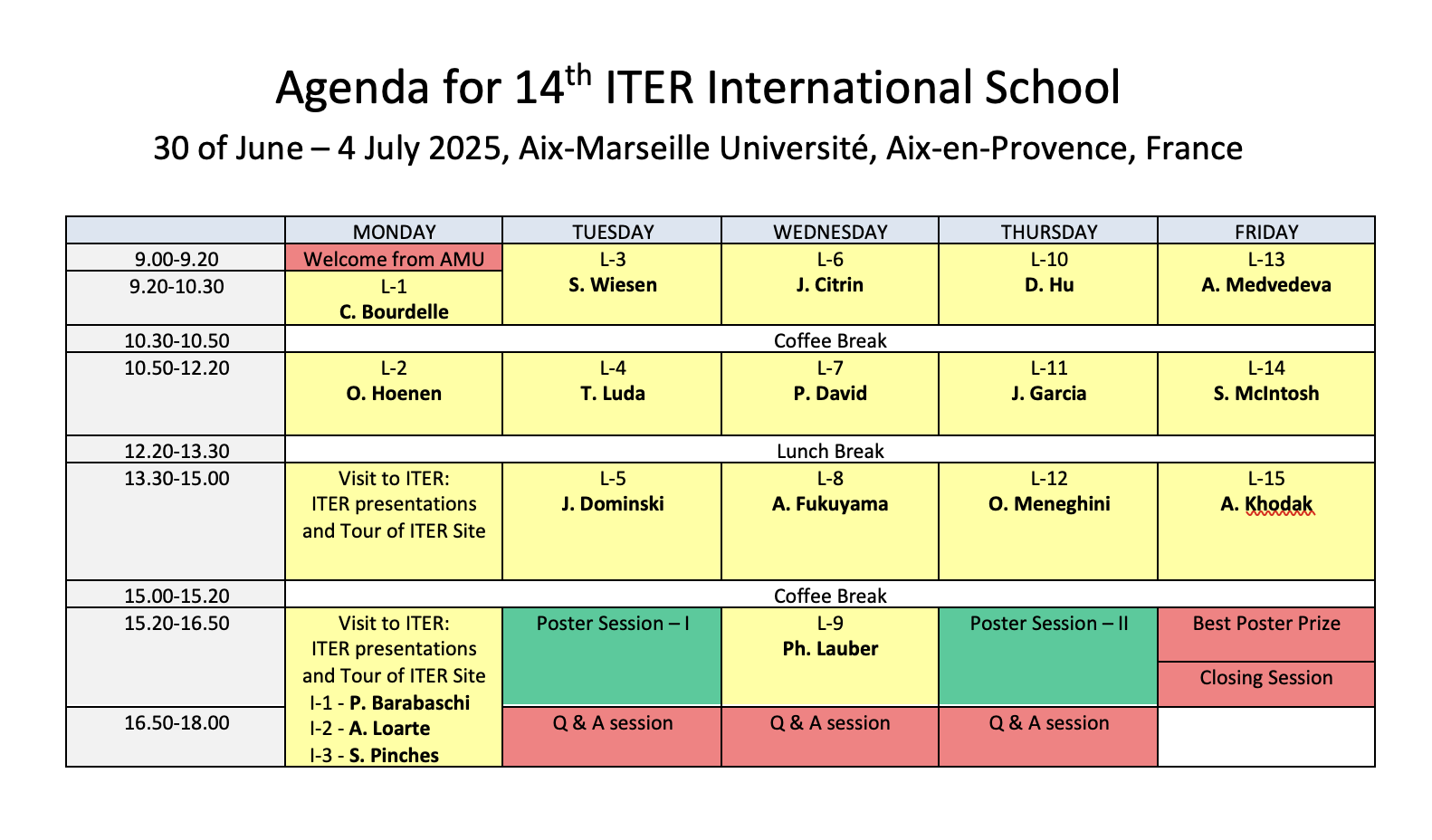
Schedule:
L-1. General Introduction to Integrated modelling. C. Bourdelle, IRFM, CEA, France
L-2. Infrastructure development for integrated modelling. O. Hoenen, ITER Organization
L-3. Integrated modelling for SOL and PWI (erosion deposition and edge plasmas). S. Wiesen, DIFFER, Nederlands
L-4. Integrated modelling for edge-core compatibility. T. Luda, Max-Planck-Institut für Plasmaphysik, Germany
L-5. Core + edge gyro-kinetic integrated simulations. J. Dominski, Princeton Plasma Physics Laboratory, USA
L-6. Application of Machine Learning for development of fast models for integrated modelling. J. Citrin, Deepmind, Google
L-7. Flight simulators (i.e. embedding integrated plasma models into plant models and control system models closing the loop for control) P. David, Max-Planck-Institut für Plasmaphysik, Germany
L-8. Integrated modelling for H&CD. Atsushi Fukuyama, QST- Kyoto University, Japan
L-9. Integrated modelling for fast particles and MHD. Ph. Lauber, Max-Planck-Institut für Plasmaphysik, Germany
L-10. Integrated modelling of disruptions (incl. mitigation, REs, etc.). Di Hu, Beihang University, China
L-11. Use of integrated modelling to plan experiments and validation in DT. J. Garcia, IRFM, CEA, France
L-12. Validation of integrated models and uncertainty quantification. O. Meneghini, General Atomics, USA
L-13. Use of integrated models to interpret measurements. A. Medvedeva, Aix-Marseille University, France
L-14. Use of integrated models to support machine assembly. S. McIntosh, ITER Organization
L-15. Use of integrated models for neutronic analysis. A. Khodak, Princeton Plasma Physics Laboratory, USA
ITER Visit Talks
1. Welcome by IO management - P. Barabaschi, Director General, ITER Organization
2. ITER status and application of integrated modelling to support design, operation and research plan. A. Loarte, ITER Organization
3. The ITER integrated modelling development programme. S. Pinches, ITER Organization
Lecture Abstracts
This page provides a brief introduction to the lectures of the 14th ITER International School. Abstracts are updated continuously as they are received from the speakers.
L-1. General Introduction to Integrated modelling.
C. Bourdelle, IRFM, CEA, France
In tokamak plasmas, a nonlinear interplay between transport and sources/sinks takes place for all transported quantities (current, heat, particle and momentum). Thanks to integrated modelling frameworks, we can iterate physics based quasilinear turbulent transport models over multiple confinement times. Such modelling allows predicting current, temperature, density and rotation profiles and disentangle the causality at play behind the modeled time evolution.
An intense validation effort of such modelling against experimental measurements has been on-going and has progressed our understanding. In dynamical phases, the so called 'cold pulse' physics has been explained in the AUG tokamak, the isotope impact in plasma current ramp up understood in the JET tokamak, the impact of the particle source (from neutral beam injection) on tungsten core accumulation clarified in JET and AUG. In stationary phases, the saturation of the ion temperature in electron heated WEST plasmas has been understood, the energy content has been predicted with higher accuracy than empirical scaling laws with respect to plasma current, magnetic field, plasma size and gas fueling; both in L and H modes on AUG.
Validation of physics based integrated modelling allows control optimization in preparation of ITER operation as well as risk reduction for future reactors design. However, despite the reported progresses, physics gaps remain on this path. For example, unlike today’s devices, ITER-class devices will be opaque to neutrals and fueled by pellets. In absence of physics understanding of the transport in the pedestal, extrapolation is uncertain. Moreover, in burning plasmas, the non-linear coupling between the central core profiles and the fusion power is very strong. The uncertainties on profile predictions due to unverified and invalidated reduced transport models in such high pressure plasmas lead to uncertain fusion power predictions. Routes on how to address these challenges within integrated modelling will be proposed.
L-2: Infrastructure development for integrated modelling.
O. Hoenen, ITER Organization
Simulating a physics phenomena using a holistic, integrated modelling (IM) approach necessitates a high level of integration between a wide range of physics knowledge, modelling codes and information (data). This may involve coupling multiple scales (in time and space), different physics (plasma-material interactions, turbulent transport, ...), different discretizations (particles, fluid descriptions) and also multiple software and computing technologies. Such an approach requires a close and sustained collaboration of inter-disciplinary experts, ideally within an open environment in order to ease communications and information sharing. In addition to this necessary convergence of expertise, existing software infrastructures and collaborative development platforms can be leveraged to support various stages of an integrated modelling effort. This lecture will present the useful computational science concepts and associated software tools to support experts in developing IM applications. After reviewing some general concepts and notions, different software engineering approaches to IM will be presented and discussed. Relevant good practices, that apply beyond IM, will also be covered. Finally, the overall concepts behind the Integrated Modelling & Analysis Suite (IMAS) at ITER will be presented. Different supporting tools in the IMAS infrastructure will be detailed and put in the context of a few chosen practical applications.
L-3: Integrated modelling for SOL and PWI (erosion deposition and edge plasmas)
Sven Wiesen, DIFFER, the Netherlands
In this lecture, the importance of integrated edge-to-core modelling to predict the operational window of a fusion reactor like ITER or DEMO is highlighted. Avoidance of excessive power loads on the plasma-facing components during transients (that also exist during the flat-top phase of a discharge e.g. during pellet ablations) is crucial and integrated models (high-fidelity flight-simulators, HFPS) like JINTRAC are already able to predict reactor scale discharge dynamical conditions. Tungsten (W) particle erosion from the divertor and first-wall plasma-wall interactions (PWI) delimits or even could inhibits good performance of the of the burning fusion plasma if not properly controlled.
A brief introduction on power and particle exhaust is given and the concept of a radiative divertor in detached conditions is introduced. The role of impurity radiation and transport at the plasma edge in fusion devices is highlighted especially for devices with a metallic wall. Some important aspects for future reactors are described with the focus on enhanced dissipation by plasma core radiation. The implications on the pedestal fuelling and core confinement are highlighted. New operational exhaust regimes like the Quasi-Continuous Exhaust (QCE) regime or the X-point radiator (XPR) are briefly discussed that could be helpful to enlarge a fusion reactor’s operational space.
Kinetic impurity erosion and transport codes like ERO2.0 have gained significant maturity of the past decade and are now regularly used to interpret W-transport in metallic devices. For consistent predictions on reactor scale, such PWI codes require validated input from plasma-edge codes like SOLPS-ITER especially for plasma flows and conditions in the region close to the wall. The amount of eroded W that reaches the core region has a direct impact on the power conditions from the core into the scrape-off layer region (SOL) and the SOL’s ability to exhaust power flows to the wall. A global integration of plasma transport and PWI models is thus mandatory to tackle the occurring interdependencies in the core, edge and wall regions.
Over the past years, the JINTRAC integrated model has undergone a continuous validation process and some examples will be demonstrated (e.g. for the JET Be/W wall). JINTRAC is now regularly being employed as an integrated edge-core model, e.g. as a predict-first tool for ITER’s full-W first-wall conditions with some success. However, a self-consistent model for W-transport from the wall through the scrape-off-layer into the core (pedestal) region is still to be validated (and especially in the far-SOL region the model uncertainty is still high). To allow for a speed-up of the validating process, fast global JINTRAC simulations are required. Recently, new numerical methods based on Machine Learning / Deep Learning (AI based) have been developed also for the SOL region. Such fast surrogate models can now be employed and integrated to replace some bottlenecks in the model pipeline of JINTRAC. An example is given for speeding up the SOL simulations by using a neural network variant of SOLPS-ITER.
L-6: Application of Machine Learning for development of fast models for integrated modelling
Jonathan Citrin, Google DeepMind
Significant progress in integrated modelling frameworks and physics models has been made in recent times, resulting in increased accuracy validated by experimental measurements. These advancements have enabled applications in experimental interpretation, multiphysics validation and understanding, and extrapolation to future performance. However, accuracy and computational tractability are conflicting constraints in traditional integrated modelling. Many use-cases require both fast and accurate simulation, particularly for many-query problems like scenario optimization and controller design.
This lecture reviews the use of machine learning to develop such fast and accurate models, as well as differentiable integrated modelling frameworks that leverage their features. Techniques discussed include ML-surrogate generation through neural network regression of higher-fidelity model databases, accelerating higher-fidelity models for practical database generation, and learning directly from data. Furthermore, the differentiability of neural network models enables differentiable simulation, unlocking nonlinear PDE solving and gradient-driven optimization applications. In this context we review modern differentiable integrated modelling solvers, focusing on the TORAX code, written with JAX, a Python library offering auto-differentiability, just-in-time compilation, and strong support for coupling ML-surrogates.
L-7: Flight simulators (i.e. embedding integrated plasma models into plant models and control system models closing the loop for control)
P. David, Max-Planck-Institut für Plasmaphysik, Germany
Future fusion devices are complex systems and present-day experimental devices need additional versatility to test as large a parameter set as possible. To that end, more and more advanced control and monitoring strategies, and (virtual) actuators, are used, such that although each element is well known, the system's behaviour exceeds the predictability of its individual components. As a result, being able to run simple yet realistic enough simulations that include the plant systems, the plasma and their interactions became particularly important. Such simulation platforms, usually referred to as flight simulators, are designed to use the experimental discharge program as input, and to output the temporal evolution of both the plant and the plasma quantities. In addition, flight simulators are expected to be used iteratively to test and improve scenarios or models. Therefore, while any integrated model is technically eligible, only simplified models remain practically viable due to the time constraints. The lecture will describe the concept of flight simulators, with a focus on the control system elements surrounding the physics model. In particular, the requirements and benefits of closed-loop simulations will be highlighted.
L-8: Core-Edge Integrated Gyrokinetic Simulations of Fusion Plasmas
J. Dominski, Princeton Plasma Physics Laboratory, USA
Modeling plasma performance in ITER is a significant challenge due to the complex interplay between the multiple species composing the plasma. A key aspect is the role of the wall and edge region, where impurities are sputtered or injected before propagating toward the core. In particular, tungsten (W) accumulation is a critical issue affecting the H-mode of operation, while low-Z impurities such as nitrogen (N) or boron (B) have been shown to mitigate this accumulation.
This lecture will present how recent gyrokinetic simulations including collisional transport, turbulence, and atomic physics are addressing these challenges through core-edge integrated modeling. An emphasis will be given on the recent efforts to achieve high-fidelity impurity transport and radiation modeling using atomic data from the ADAS database. Validation of these models against plasmas of the WEST tokamak will be presented and whole-device XGC simulations of an H-mode plasma, incorporating multiple impurity species in ASDEX-U, will be presented, showcasing new capabilities for studying tungsten screening and pedestal physics. Finally, we will explore different approaches for capturing the impact of edge physics on core profiles, including global and local gyrokinetic models coupled to transport codes.
L-9: Integrated modelling for fast particles and MHD
Ph. Lauber, Max-Planck-Institut für Plasmaphysik, Germany
In this lecture the challenge of energetic particle (EP) transport in hot fusion plasmas will be discussed. After briefly repeating a few basics about EP sources and related distribution function properties, various transport mechanisms are introduced: transport induced by static field perturbations, low-frequency MHD modes and various branches of Alfven and kinetic Alfven waves. Next, the most important presently existing models and their ingredients are reviewed: critical gradient model, kick model, resonance broadening model, flux matching models and the phase space zonal structure model. Their differences and range of applicability are demonstrated using examples from present-day devices and future ITER predictions. By comparing with transport models for the thermal background, the multi-scale nature of the problem - introduced by EPs - is highlighted. Direct and indirect cross-scale coupling effects are summarised, and very recent strategies to capture their impact on the overall transport predictions conclude the lecture.
L-10: Integrated modelling of disruptions and their mitigation
Di Hu, Beihang University, China, and the JOREK team
Disruptions consist of the sudden and global loss of confinement in magnetically confined plasmas. During disruptions, thermal and magnetic energies of the plasma tend to deposit on the Plasma Facing Components (PFCs) asymmetrically, resulting in localized load that would cause severe damage to the device. Such damage ranges from PFC melting and coolant leakage induced by localized heat flux or runaway electron impact, to mechanical damage by the electro-magnetic (EM) load. For future high-performance devices such as ITER or DEMOs, such damage could severely reduce the lifetime of the machine components and cause operational delays. Hence the understanding and mitigation of disruptions are of utmost importance.
Due to the multi-scale physics nature of disruptions, integrated modelling of disruptions and their mitigation is crucial, as their various physical aspects are intrinsically coupled and not separable. For example, the fast-moving, relativistic runaway electrons (REs) generated during disruptions could carry significant amount of current and affect the relatively slowly evolving MHD fluid instabilities, while the strong MHD instabilities would in turn affect the RE transport and deposition as the magnetic field lines become stochastic. Another example: atomic physics of neutrals, impurities and radioactive isotopes, either intrinsic or induced during the disruption mitigation, would have strong impact on the global MHD instability and the RE generation, which in turn would influence the local ionization, recombination and radiation of the said species. The list goes on.
Meanwhile, Disruption Mitigation Systems (DMS) such as the Shattered Pellet Injection (SPI) has been developed for ITER. However, the mitigation of all disruption consequences simultaneously could be extremely challenging as their optimization condition could be contradictory, and the mitigation configuration space is multi-dimensional. All these inter-linked, multi-scale physics call for the challenging yet necessary integrated 3D modelling effort.
Simulation tools such as the 3D nonlinear code JOREK, used together with the Integrated Modelling & Analysis Suite (IMAS) platform, have been developed to model disruption and its mitigation to improve the disruption mitigation efficiency. One example of such modelling is the coupling between the JOREK reduced MHD fluid simulation with the particle simulation of REs to simulate the formation of the RE current plateau and its suppression through MHD instabilities. Another example is the coupling between the MHD simulation, the pellet ablation module and the atomic physics module to find the most efficient SPI configuration. Yet more physics modules could be included such as the sputtering and plasma-wall current interaction. Together, the integrated modelling of all these components enable high-fidelity simulation of disruptions and their mitigation in contemporary and future tokamaks, paving the road to successful disruption control.
L-11: Use of integrated modelling to plan experiments and validation in DT.
J. Garcia, IRFM, CEA, France
For more than a decade, an unprecedented predict-first activity has been carried in order to predict the fusion power and provide scientific guidance to the second Deuterium–Tritium (D–T) campaign performed at JET in 2021 (DTE2). Such an activity provided a framework for a broad model validation and development towards the D–T operation. It is shown that it is necessary to go beyond projections using scaling laws in order to obtain detailed physics based predictions. Furthermore, mixing different modelling complexity and promoting an extended interplay between modelling and experiment with different hydrogen isotopes are essential towards reliable predictions of D–T plasmas. The fusion power obtained in this predict-first activity was in broad agreement with the one finally measured in DTE2, however drawbacks and flaws in the present-day modeling capabilities were also found. The profound implications for the prediction of fusion power in future devices will be discussed. In particular, the influence of this predict first activity on the development of the ITER research plan will be highlighted.
L-13: Use of integrated models to interpret measurements
A. Medvedeva, Aix-Marseille University, France
Understanding magnetic confinement fusion plasmas requires accurate interpretation of experimental measurements, which are inherently indirect and influenced by multiple intertwined physical processes. Extracting meaningful insights demands a robust comparison with experiments through an integrated modeling approach that accounts for both core and edge plasma while incorporating transport, turbulence, and impurity physics.
This lecture will explore how synthetic diagnostics serve as a crucial link between simulations and experiments, enabling direct comparison of model predictions with measured signals. By replicating real diagnostic observations, synthetic diagnostics help identify the dominant physical mechanisms shaping experimental data. Embedding them within integrated modeling frameworks allows for a systematic assessment of transport assumptions, turbulence characteristics, and impurity contributions, improving the reliability of model validation.
We will present case studies from tokamak experiments, illustrating how integrated modeling enhances measurement interpretation while addressing challenges such as diagnostic uncertainties, model fidelity, and computational constraints. The role of synthetic diagnostics in refining measurement interpretations, optimizing diagnostic configurations, and improving predictive capabilities for reactor-scale plasmas will also be examined. The lecture will conclude with perspectives on advancing integration between modeling and diagnostics to support the next generation of fusion research.
L-15: Use of integrated models for neutronic analysis
A. Khodak, Princeton Plasma Physics Laboratory, USA
Deuterium-Tritium (DT) fusion, considered in many fusion devices including ITER, creates neutrons with a mean energy of 14 MeV. The neutrons are not confined by the magnetic field and escape the plasma confinement system. Neutrons directly affect the machine components and interact with structural materials producing secondary gammas. Several metrics need to be determined to ensure reliable operation of the systems surrounding the vacuum vessel, including neutron flux and fluence, displacements-per-atom (DPA) and energy deposition or heating. Integrated models for neutronic analysis combine various computational tools to simulate the complex behavior of neutrons within the fusion device such as ITER. These models combine neutron transport, fuel performance, thermal hydraulics, and reactor physics to provide a comprehensive framework for analyzing complex reactor systems.
This presentation explores the current state of integrated neutronic models, highlighting advancements, challenges, and future directions for improving the predictive capabilities necessary for successful fusion energy demonstration. As an example, virtual prototyping system for tritium breeding blankets, which is currently under development at PPPL uses device equilibrium to create three dimensional neutronic source distribution, which is then transferred to a Monte-Carlo neutronic analysis code. Results of neutronic analysis in the form of volumetric heat and tritium source distribution are provided to 3D Computational Fluid Dynamics (CFD), Magneto Hydrodynamics (MHD) analysis where detailed conjugate heat and mass transfer analysis is performed. Results of CFD analysis in the form of pressure and temperature distributions together with DPA data from neutronic analysis are then supplied to a structural code for 3D non-linear structural analysis, which includes nuclear swelling.